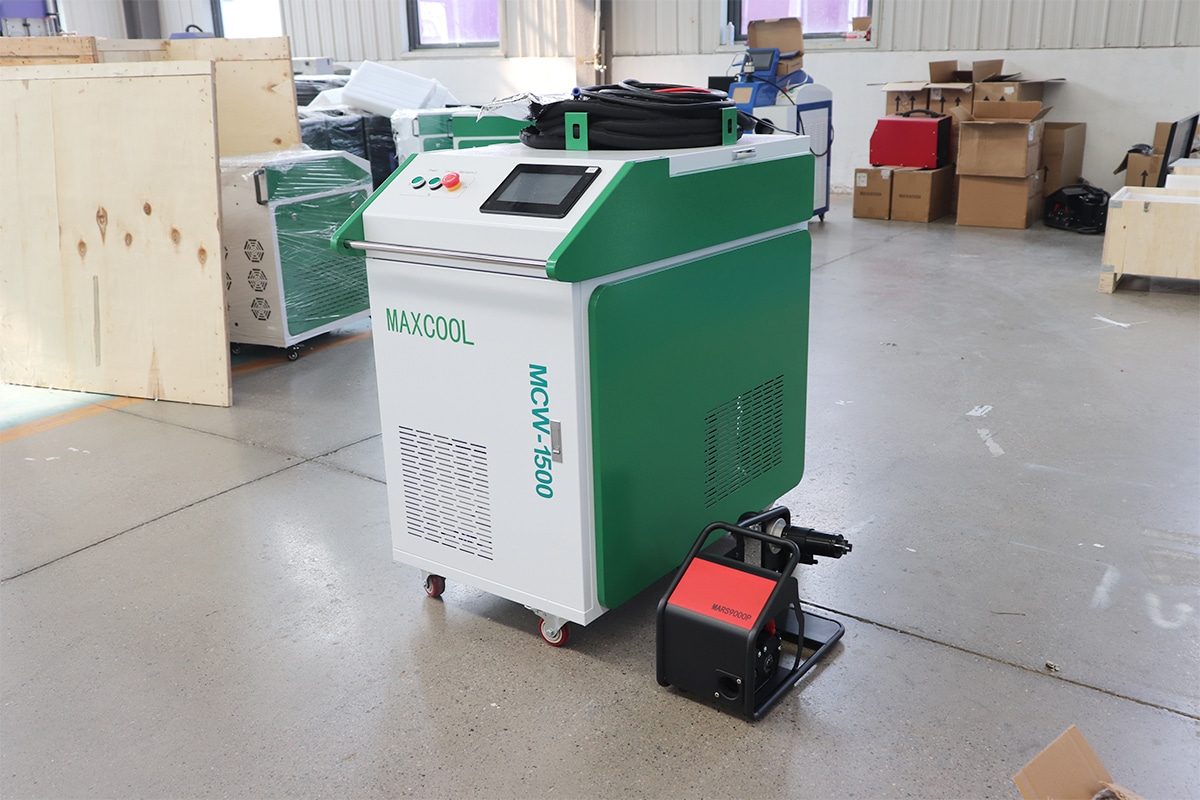
What is Laser Welding?
Laser welding is a fusion welding process that utilizes a highly concentrated laser beam to heat and join materials. The process is renowned for its ability to create strong, precise welds in metals, plastics, and even dissimilar materials. There are two primary modes of laser welding:
- Conduction Mode Welding: This method relies on surface melting, where the laser energy is absorbed and distributed across the surface. It is ideal for thin materials and applications requiring smooth, aesthetically pleasing welds.
- Keyhole Mode Welding: In this mode, the laser creates a small, vaporized cavity (keyhole) in the material. This enables deeper penetration and is suitable for thicker materials.
The benefits of laser welding include:
- High-speed operation, reducing production time.
- Minimal heat-affected zones (HAZ), preserving material properties.
- Flexibility to weld intricate geometries and small components.
- Compatibility with automation for enhanced productivity.
Factors Affecting Laser Weldability
Material Properties
Material Composition
- Homogeneous Materials: Pure metals like titanium, stainless steel, or low-carbon steel are easier to weld because they have consistent physical properties.
- Alloys: Aluminum alloys and magnesium alloys often face challenges like hot cracking due to the presence of low melting point phases. Alloying elements such as zinc and silicon can significantly alter the behavior of the weld pool.
- Dissimilar Materials: Welding dissimilar materials, like aluminum to steel, requires special considerations such as the use of intermediate layers or filler materials to reduce intermetallic compound formation.
Thermal Conductivity
- High Conductivity Materials: Copper and aluminum dissipate heat rapidly, requiring higher laser power to achieve sufficient penetration.
- Low Conductivity Materials: Materials like stainless steel and titanium concentrate heat in the weld zone, making them easier to process with lower power.
Reflectivity
- Reflective materials (e.g., aluminum, copper, and gold) reflect a significant portion of laser energy, leading to reduced efficiency. Solutions include:
- Pre-coating the surface to enhance absorption.
- Using shorter wavelengths, such as from fiber lasers, to increase absorption efficiency.
Melting Point
- Materials with high melting points (e.g., tungsten) require more energy and precise focus.
- Conversely, low-melting-point materials (e.g., lead) risk burn-through and excessive evaporation, necessitating tight parameter control.
Absorption Characteristics
- Different wavelengths are absorbed differently by materials. Metals typically absorb fiber laser wavelengths (1.06 µm) more effectively than CO2 laser wavelengths (10.6 µm).
Surface Condition
- Contaminants: Dirt, grease, or oxide layers can introduce defects like porosity or weak bonds.
- Surface Finish: A rough surface scatters the laser beam, reducing energy absorption. Polished or prepared surfaces improve energy concentration.
Laser Parameters
Laser Power
- Low Power: Suitable for thin materials or applications requiring fine details.
- High Power: Necessary for thick materials or high-speed applications, but excessive power can cause defects like spatter and keyhole instability.
Laser Wavelength
- Fiber lasers with shorter wavelengths are ideal for metals. CO2 lasers with longer wavelengths are effective for non-metals and plastics.
Beam Spot Size
- A smaller beam spot increases energy density, leading to deeper penetration and narrower welds, while a larger spot is preferred for broader seams.
Welding Speed
- Faster speeds reduce heat input, minimizing distortion, but may lead to incomplete fusion. Slower speeds increase heat input, enhancing penetration but raising the risk of thermal damage.
Focus Position
- Correct positioning ensures maximum energy delivery to the weld zone.
- Above the surface: Wider but shallower welds.
- Below the surface: Deeper penetration.
Pulse Duration
- Short pulses are ideal for small, precise components or heat-sensitive materials. Longer pulses ensure deeper penetration for thicker materials.
Joint Design
Joint Type
- Butt joints are easy to weld with minimal preparation.
- Lap joints require careful parameter control to prevent heat buildup in the overlapping region.
- T-joints and edge joints are used for specific geometries and require precise alignment.
Joint Preparation
- Proper cleaning and alignment are critical. Impurities or poor alignment lead to defects such as porosity or weak bonds.
Material Thickness
- Thin materials need low power to avoid burn-through. Thicker materials may require multiple passes or preheating.
Gap Tolerance
- Excessive gaps weaken the weld. Using filler materials or tighter tolerances can improve results.
Environmental Factors
Shielding Gas
Protects the weld pool from oxidation and contamination. Common gases include:
- Argon: Versatile and cost-effective.
- Helium: Promotes deeper penetration but is more expensive.
- Nitrogen: Occasionally used but may introduce nitrides in some metals.
Ambient Conditions
- Dust, humidity, or extreme temperatures can disrupt weld quality. Controlled environments, such as clean rooms, are ideal.
Stability
- Vibrations or movement can misalign the laser beam, causing defects. Using stable fixtures minimizes such issues.
Process Monitoring and Control
Process Automation
- Automated systems provide repeatability and reduce human error. Robotic arms ensure consistent speed and alignment.
Real-Time Monitoring
- Sensors detect defects or inconsistencies during welding, enabling immediate adjustments to parameters.
Quality Control
- Non-destructive testing methods like ultrasonic or X-ray inspections verify weld integrity.
Operator Expertise
Training
- Operators must understand material properties, laser parameters, and safety protocols.
Experience
- Experienced operators can quickly identify and rectify issues such as cracking or porosity.
Post-Welding Considerations
Cooling
- Controlled cooling minimizes residual stresses and distortion.
Heat Treatment
- Improves mechanical properties and relieves stress.
Inspection and Testing
- Methods like visual inspection, ultrasonic testing, or tensile testing ensure weld strength and durability.
Strategies to Improve Material Weldability
Surface Preparation
Proper surface preparation is one of the simplest yet most effective ways to improve material weldability:
- Cleaning: Remove contaminants like dirt, oil, and grease using chemical cleaning agents or ultrasonic cleaners. This ensures a clean weld pool free of impurities.
- Oxide Removal: Use mechanical methods (grinding or brushing) or chemical treatments (acid etching) to eliminate surface oxides that reduce energy absorption.
- Surface Coating: For reflective materials like aluminum or copper, applying a temporary coating (e.g., a thin layer of graphite or black paint) enhances laser energy absorption.
Use of Filler Materials
Filler materials can bridge the gap between dissimilar materials or enhance weld properties:
- Dissimilar Material Welding: Use a compatible filler alloy to minimize the formation of brittle intermetallic compounds, especially when welding metals like aluminum and steel.
- Mechanical Reinforcement: Filler wires can improve joint strength and reduce stress concentrations in high-load applications.
Optimization of Laser Parameters
Precise control of laser parameters ensures optimal energy delivery and minimal defects:
- Laser Power: Calibrate the power level to match the material’s thermal conductivity and thickness.
- Welding Speed: Experiment with different speeds to balance penetration depth and heat input.
- Beam Focus: Use advanced focusing optics to maintain a consistent energy density, even for complex geometries.
Use of Advanced Shielding Techniques
Shielding gas is vital for protecting the weld pool and improving weld quality:
- Customized Gas Mixtures: Experiment with blends of argon, helium, and nitrogen for specific materials.
- Gas Flow Optimization: Ensure a laminar flow over the weld pool to avoid turbulence and porosity.
- Dual Gas Shielding: For highly reactive materials like titanium, use an inner gas layer to protect the weld zone and an outer layer to shield surrounding areas.
Heat Management
Managing heat input and dissipation is critical for weld quality:
- Preheating: Preheating high-conductivity materials like aluminum or copper reduces the thermal gradient and improves fusion.
- Controlled Cooling: Use cooling fixtures or controlled environments to minimize residual stresses and prevent cracking.
Process Innovation
Adopting innovative laser welding techniques can significantly enhance weldability:
- Hybrid Laser Welding: Combining laser welding with another process like MIG or TIG welding improves flexibility and reduces defects.
- Beam Shaping: Advanced beam shaping technologies allow for better control over energy distribution, especially for dissimilar materials.
- Adaptive Control Systems: Real-time sensors and AI-driven control systems adjust laser parameters dynamically for consistent quality.
Common Challenges of Laser Welding
Porosity
Causes:
- Insufficient shielding gas coverage.
- Rapid solidification trapping vaporized material.
- Surface contaminants release gases during welding.
Solutions:
- Use high-purity shielding gases and optimize their flow rate.
- Ensure proper joint preparation, including degreasing and oxide removal.
- Adjust laser power and speed to allow gases to escape before solidification.
Cracking
Causes:
- Thermal stresses during rapid cooling.
- Segregation of low-melting-point elements in alloys.
Solutions:
- Preheat the material to reduce thermal gradients.
- Use filler materials to improve joint flexibility.
- Optimize cooling rates to avoid excessive stress.
Reflectivity and Energy Efficiency
Causes:
- The reflection of laser energy reduces process efficiency.
Solutions:
- Use shorter wavelength lasers (e.g., fiber lasers) for better absorption.
- Pre-treat the material surface with absorptive coatings.
Incomplete Fusion
Causes:
- Insufficient laser power or incorrect focus position.
- Excessive welding speed.
- Poor joint preparation or large gaps between components.
Solutions:
- Increase laser power or adjust the focus position to ensure full penetration.
- Reduce welding speed for better energy delivery.
- Ensure precise joint alignment and fit-up.
Spatter
Causes:
- Excessive laser power or unstable keyhole formation.
- Contaminants in the weld area.
Solutions:
- Reduce laser power or use pulsed mode operation.
- Ensure clean surfaces and optimize shielding gas flow.
Distortion
Causes:
- High heat input causes expansion and contraction of the material.
Solutions:
- Use low heat input by optimizing welding speed and power.
- Secure materials with clamps or fixtures to minimize movement during welding.
Welding Dissimilar Materials
Causes:
- Differences in thermal expansion coefficients.
- Metallurgical incompatibility leading to brittle weld zones.
Solutions:
- Use intermediate layers or compatible filler materials.
- Optimize laser parameters to minimize the heat-affected zone.
Safety Concerns
Causes:
- Exposure to high-energy lasers and hazardous materials.
Solutions:
- Implement robust safety measures, including protective eyewear and ventilation systems.
- Train operators in laser safety protocols.